The PICOSEC Micromegas detector combines a Cherenkov radiator, a photocathode and a Micromegas-based amplification stage into a high-precision timing detector. Incoming particles create Cherenkov light in the radiator, which is converted to primary photoelectrons by the photocathode. These electrons are pre-amplified in a drift region and finally amplified by the Micromegas. A time resolution of 24 ps for Minimum Ionising Particles (MIPs) has been measured in several test beam campaigns in recent years and reported in NIMA 903 (2018) 317-325.
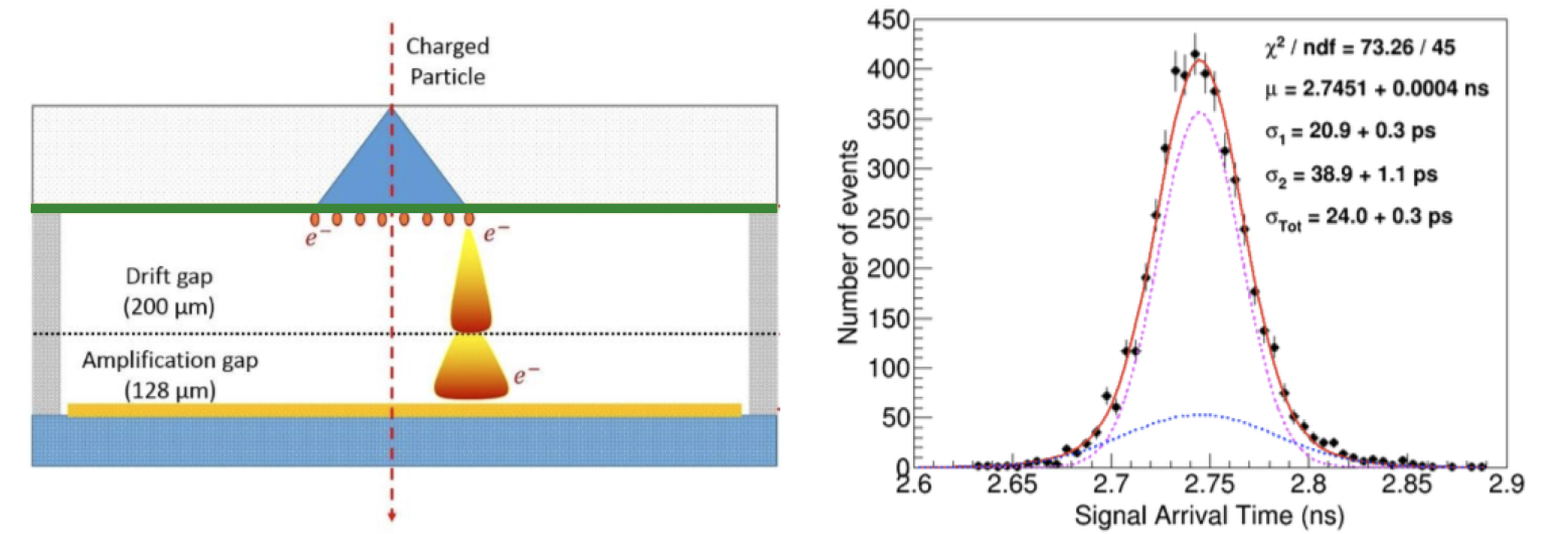
Based on successful MIP test beam campaigns and single-photon response measurements of small prototypes, the RD51 PICOSEC Micromegas collaboration is working towards large-scale, robust and radiation-hard detectors which maintain the excellent time resolution and meet future experimental requirements. Within the GDD group, we have previously developed a multi-pad prototype to study the effect of signal sharing across multiple channels in a medium-size prototype. This prototype has achieved a time resolution of 31 ps for signals shared across three neighbouring pads and is shown on the left in the figure below. We are currently developing a new PICOSEC detector module which can tiled to instrument larger areas.
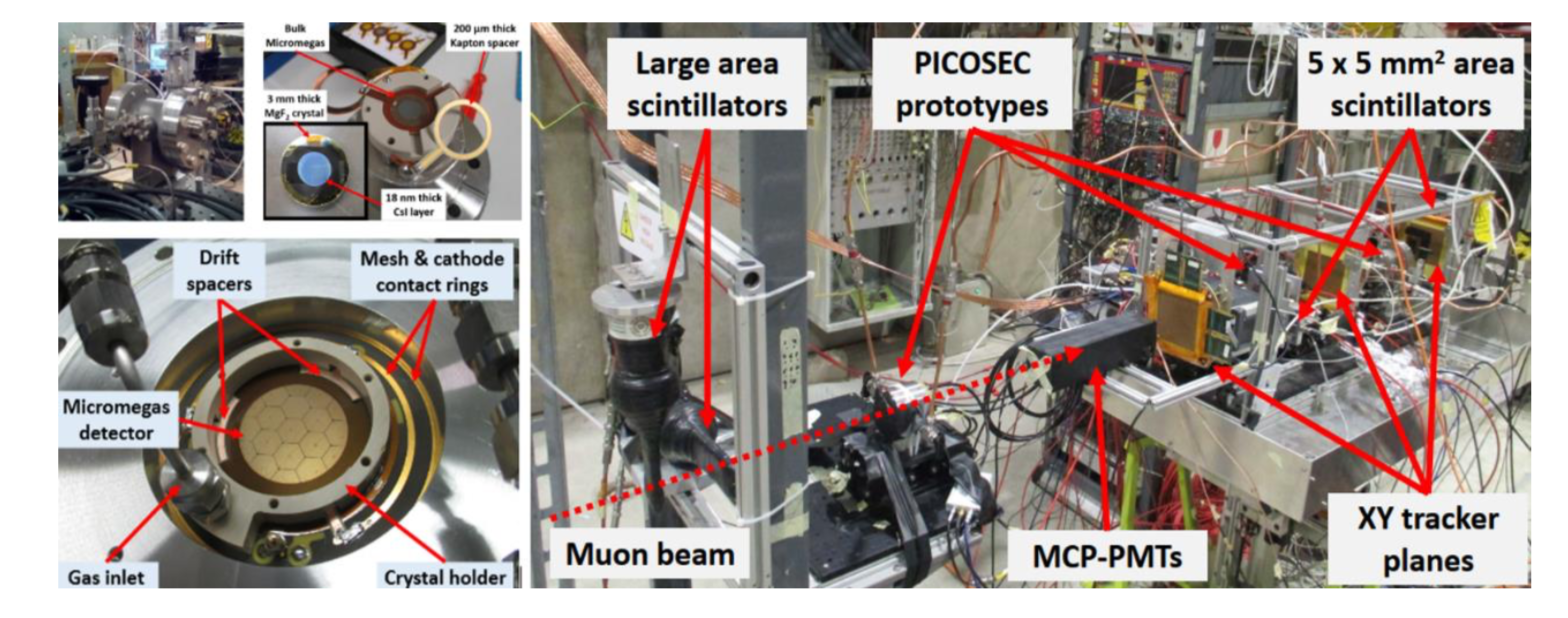
In addition to scaling up to large-area coverage, the robustness and radiation-hardness of the detector are crucial performance aspects for prolonged operation. Especially the photocathode as site of primary electron production from the Cherenkov light is important to maintain good timing performance. Ion backflow from the amplification stage onto the photocathode may damage and degrade it and destructive effects have been observed for CsI photocathodes, which have been the baseline choice owing to their high quantum efficiency (QE).
For systematic studies of alternative photocathode materials or potential protection mechanisms against ion-induced degradation, we have built the ASSET setup for wavelength-resolved measurements of the QE of photocathodes in transmission and reflective mode operation, which is shown in the central figure. This setup allows ion bombardment of photocathodes with well-defined amounts of charge and thus a quantification of the QE degradation as a function of accumulated charge. We performed systematic QE studies of a range of photocathode materials from collaborators in the PICOSEC collaboration including CsI, Diamond-Like Carbon (DLC), B4C and hydrogenated nano diamonds (HND). Preliminary results show a significantly slower degradation of QE with accumulated charge for some materials as shown in the right plot at the expense of lower initial QE compared to CsI. Further studies will focus on the quantification of QE degradation as well as the characterisation of thin protective layers on photocathodes with the ASSET setup.:
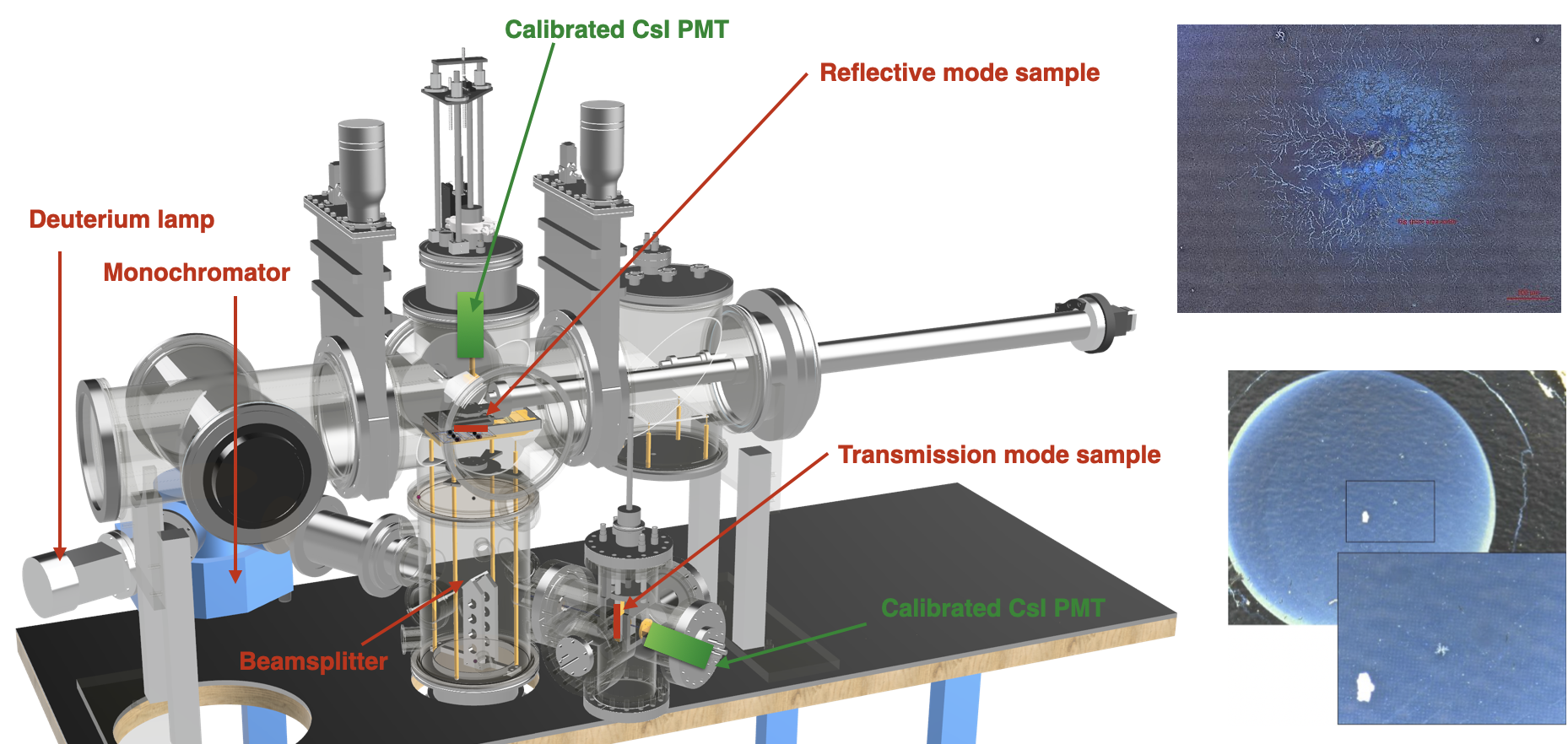