Optical readout of gaseous detectors relies on recording the scintillation light emitted during avalanche multiplication with imaging sensors. State-of-the-art CCD or CMOS cameras feature imaging sensors with a high pixel count and low noise characteristics and enable highly granular readout.
Scintillation light is emitted both during the initial interaction of incident radiation in the gaseous medium as well as during avalanche multiplication. The so-called primary scintillation light emitted during initial ionisation processes is of low intensity is therefore challenging to record with imaging sensors. The secondary scintillation light emitted during avalanche multiplication in MPGD structures benefits from the high gain factors achievable with gaseous detectors and can thus be of significant intensity.
A schematic of an optically readout out triple-GEM-based detector is shown in the left figure below. A camera is placed outside of the gas volume facing the amplification stage. Secondary scintillation light can reach the camera through a transparent viewport and is recorded by the camera.
To be recorded by the camera, the emission spectrum of the scintillation light must match the quantum efficiency of the imaging sensor. While the emission spectrum of most gases typically used in gaseous detectors is in the ultraviolet (UV) region, most cameras are focused on the visible (VIS) wavelength region. To overcome this, wavelength shifters could be used, which absorb UV light and emit VIS light. Alternatively, the addition of Tetrafluormethan (CF4) to gas mixtures can produce emission spectra which feature VIS emission bands and are therefore directly compatible with standard imaging sensors. CF4 is used in mixtures with noble gases such as Ar, He or Ne and some exemplary emission spectra are shown in the right figure below.
The broad emission bands in the UV and VIS regions are attributed to CF4, while the sharp emission lines are characteristic of the noble gases used. As a comparison, the quantum efficiency of a CCD sensor is shown by the dashed line to be well-matched to the VIS emission band of CF4 around 630 nm.
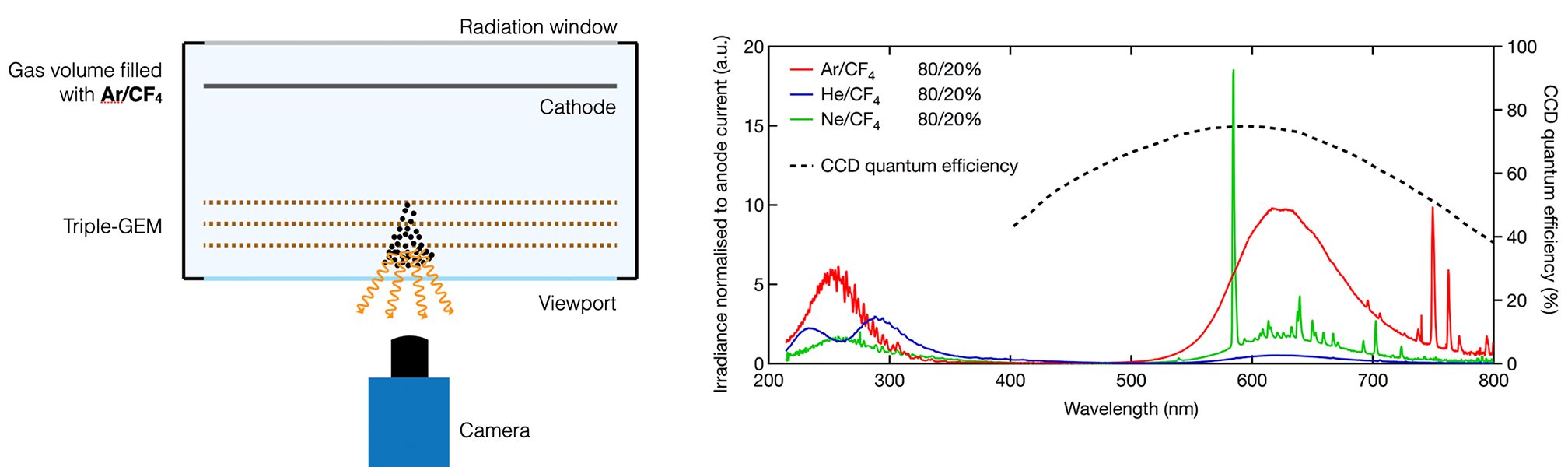
Optical readout offers an intuitive readout and 2D images acquired by the camera can be viewed on a computer in real time without the need for extensive image reconstruction. This simplifies the visualisation of radiation events, particle tracks, beam profiles as well as radiation imaging. It provides a number of advantages including the following:
- Intuitive, integrated readout approach with direct display of acquired images.
- High-granularity and good spatial resolution owing to the high pixel count of state-of-the art imaging sensors.
- Possibility to adjust the field of view and recorded active area with lenses and optics
- Placement of recording device outside of gas volume and insensitivity to electronic noise
On the contrary, several disadvantages and limitations of optical readout include:
- Limited frame rate resulting in integrated imaging approach for most CCD and CMOS cameras.
- Need for wavelength shifters or admixtures such as CF4 for light spectrum compatible with quantum efficiency of imaging sensors.
- Limited radiation hardness of imaging sensors.
We have studied the capabilities of optical readout of MPGDs for a variety of applications and developed detector taking advantage of the unique possibilities offered by this readout approach. This includes radiation imaging, X-ray radiography and fluorescence, 3D track reconstruction in optical TPCs as well as low material budget beam monitoring. These developments of optically read out GEM-based detectors along with the development of an optically read out Micromegas-based detector are shown in the sections below.
Radiation imaging
Low frame
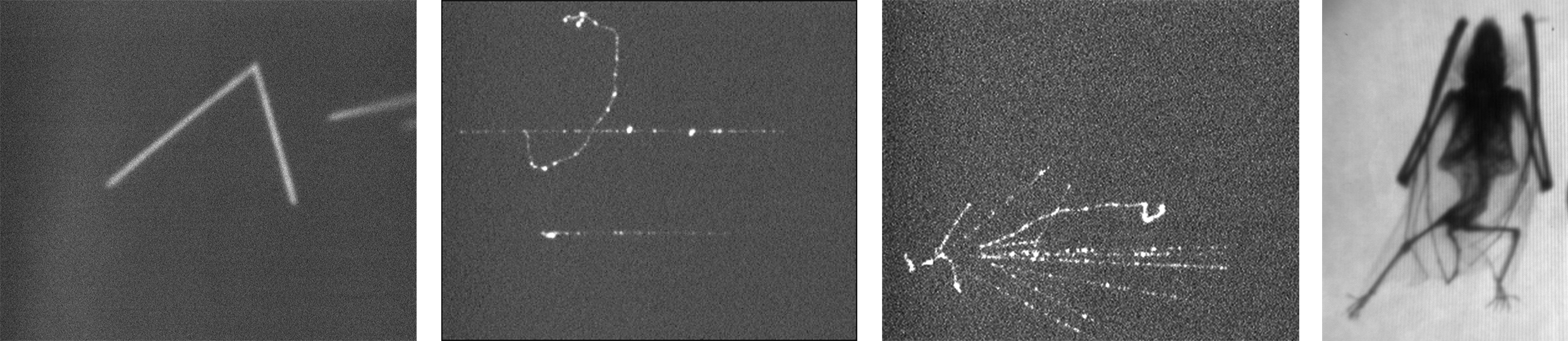
X-ray fluorescence: energy-resolved imaging
Low frame
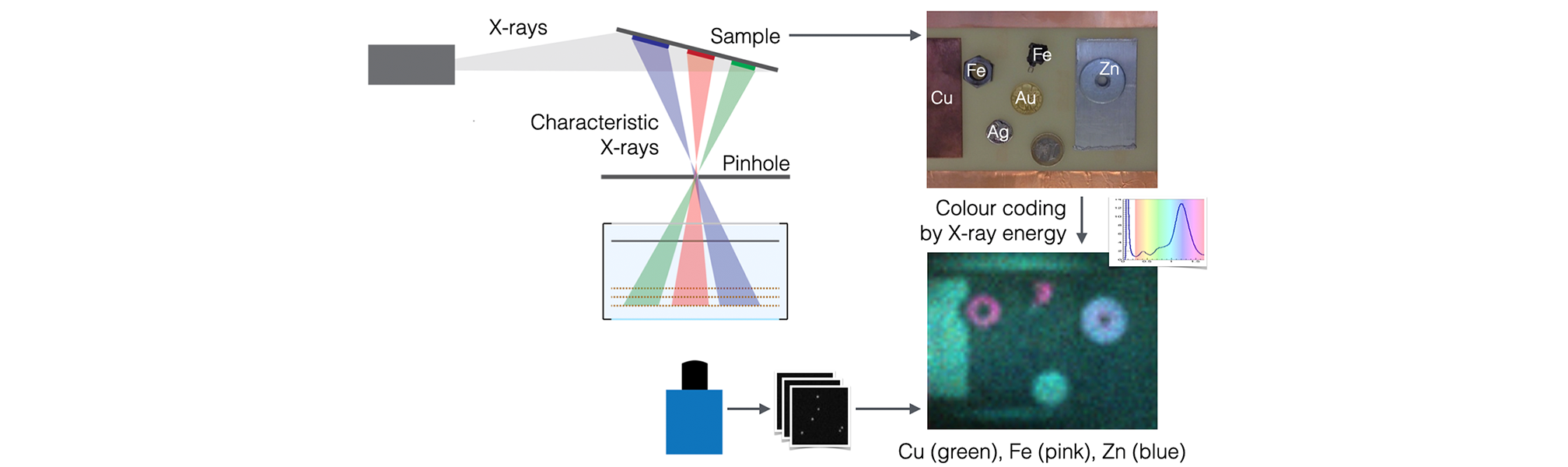
Optically read out Time Projection Chamber (TPC)
Low frame
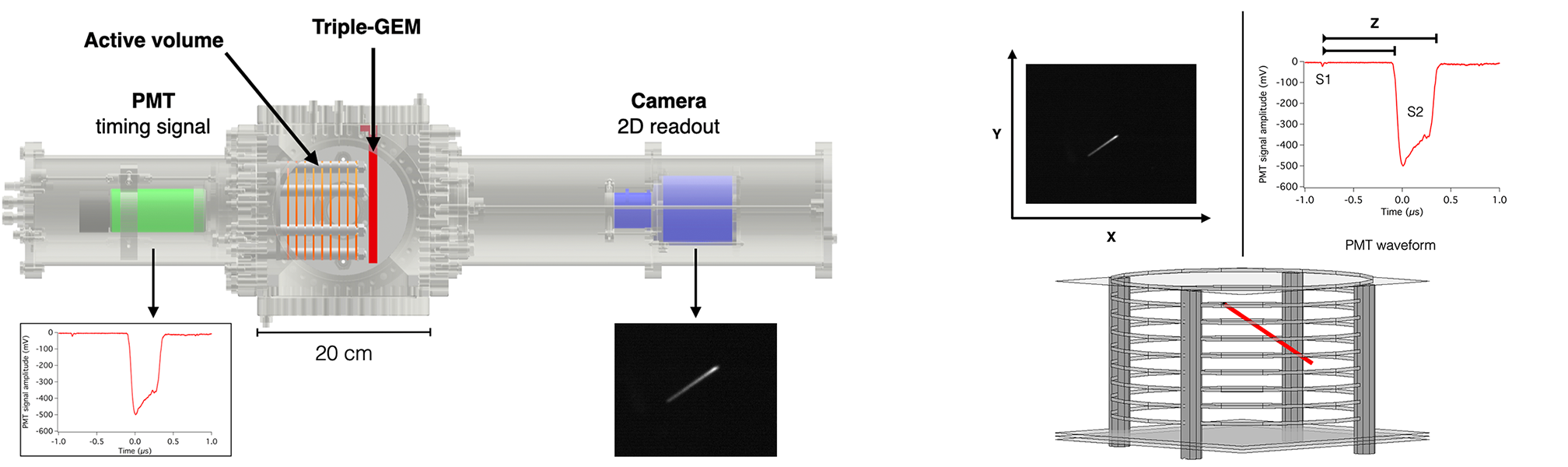
Simultaneous optical and electronic readout
Low frame
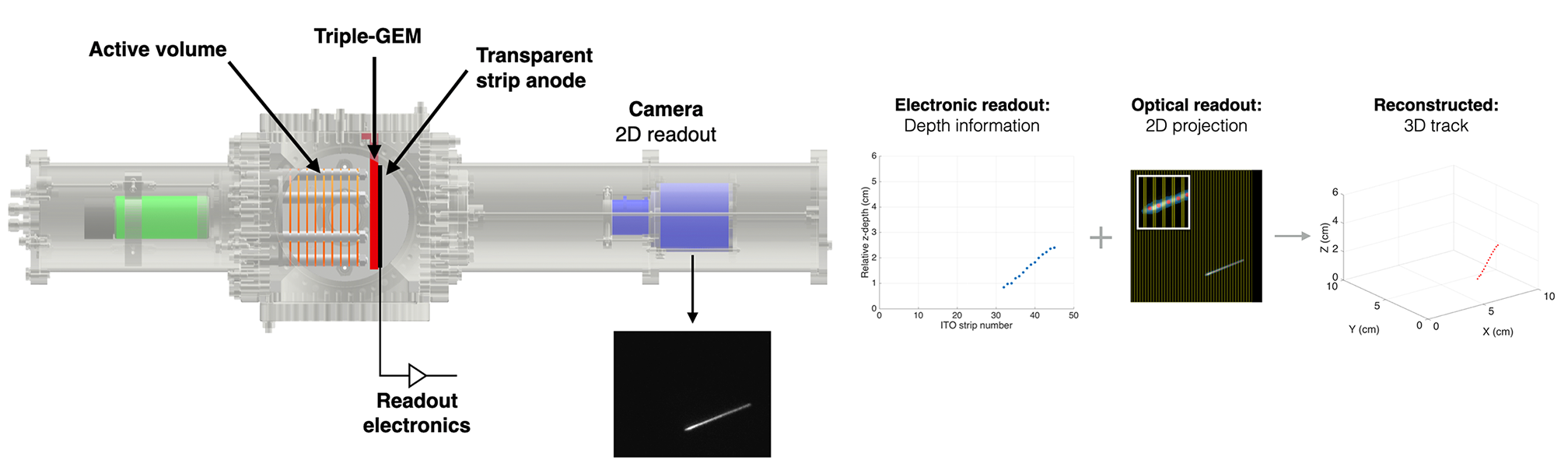
Low-material-budget dose imaging and beam monitoring
Low frame
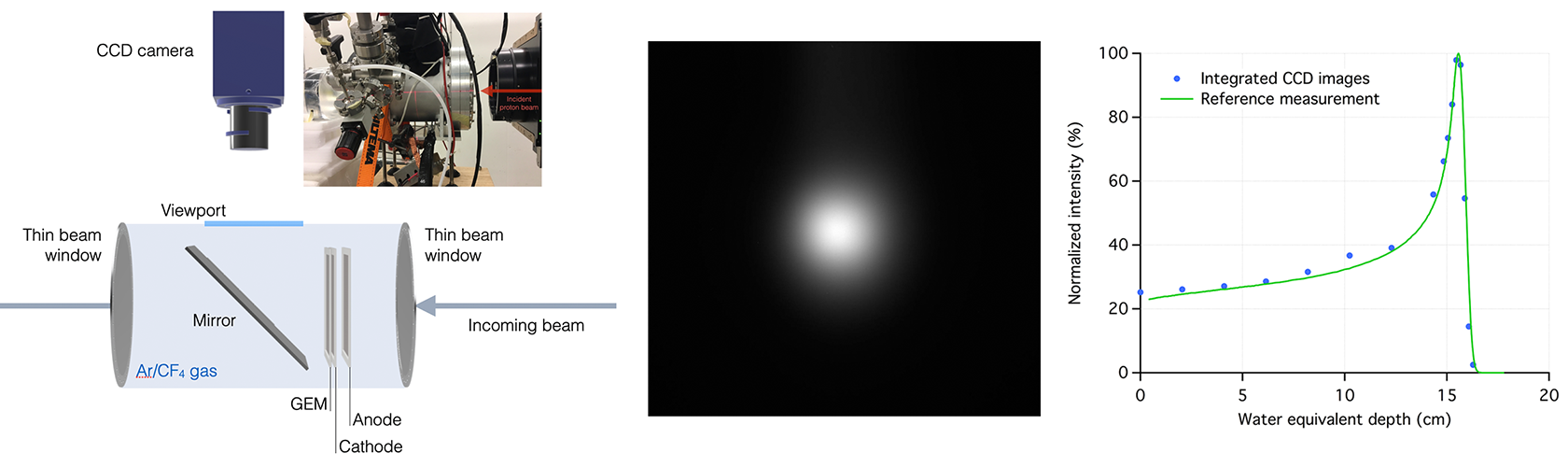
Ultra-fast optical readout
Low frame rates on the order of tens to hundreds of frames per second have previously limited optical readout of gaseous detectors to an integrated imaging approach, which is suitable for imaging applications but which is too slow for real-time beam monitoring or rapid full-field X-ray fluoroscopy. To overcome this limitation, we are working with an ultra-high-speed CMOS camera to record scintillation light from GEM-based detectors with frame rates up to one million frames per second. The high frame rates and the amount of image data recorded makes sequential acquisition, storage and off-line analysis impractical and requires the use of a streaming camera with real-time feature extraction, data reduction and online image analysis. We are studying different approaches to deal with the high data rates provided by ultra-high-speed streaming cameras including FPGA-based image filtering, GPU-based feature extraction and multi-threaded CPU image analysis algorithms to realise a continuously running optical readout system. Individual low-energy X-ray photon events have been recorded from a GEM-based detector with an ultra-high-speed CMOS camera. The acquired images were streamed to frame-grabber boards, filtered and events were extracted and used to assemble images sustaining high frame rates.
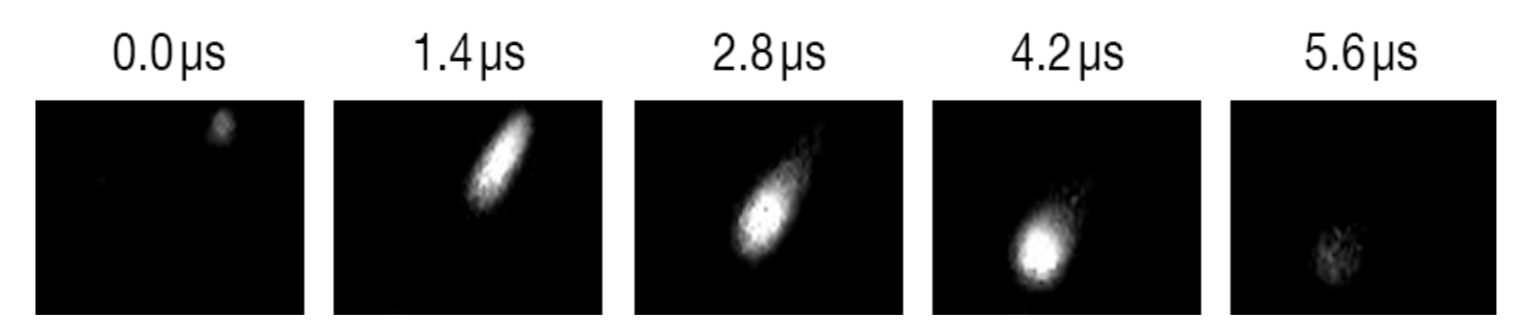
Optically read out MicroMegas
Gaseous Electron Multipliers (GEMs) are well suited for optical readout due to their geometry while most other Micro-Pattern Gaseous Detector (MPGD) varieties are integrated on opaque substrates inhibiting scintillation light readout. In collaboration with CEA/IRFU, we have demonstrated that a MicroMegas detector integrated on a glass substrate with a transparent indium tin oxide layer used as anode can be optically read out. Using this glass-based MicroMegas detector for X-ray radiography as shown in the left figure below, high spatial resolution was achieved. In addition, the good energy resolution obtained might make this detector applicable for energy-resolved imaging.
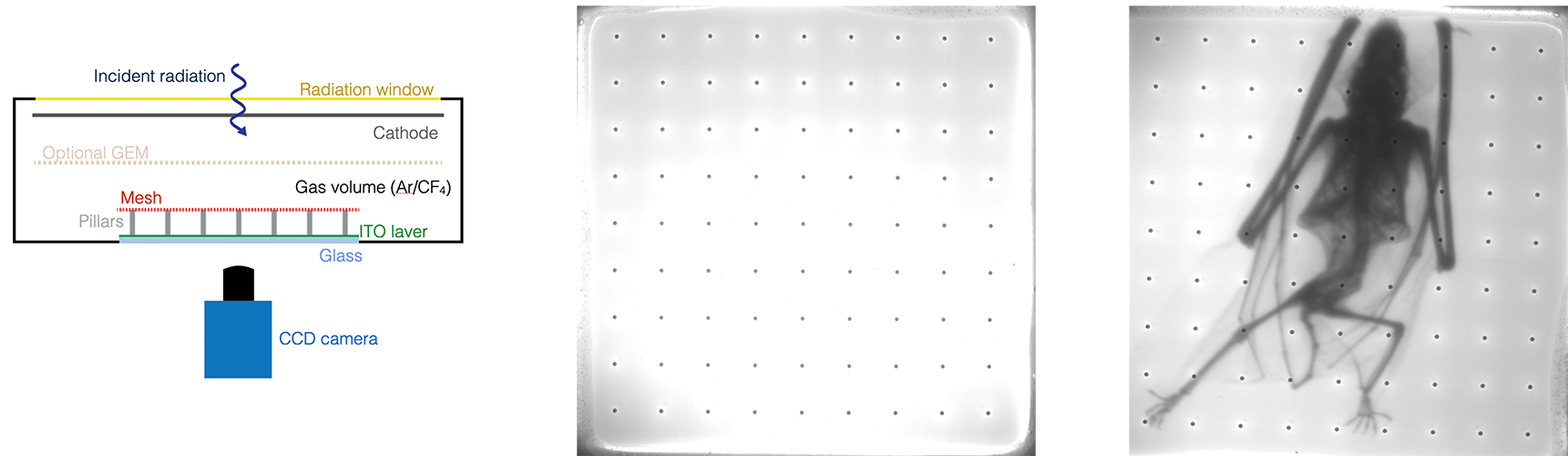
Publications
- F.M. Brunbauer et al. Radiation imaging with glass Micromegas, NIM A, 2020
- F.M. Brunbauer et al. Combined Optical and Electronic Readout for Event Reconstruction in a GEM-Based TPC, IEEE TNS, 2018
- F.M. Brunbauer et al. Live event reconstruction in an optically read out GEM-based TPC, NIM A, 2018
- F.M. Brunbauer et al. Radiation imaging with optically read out GEM-based detectors, JINST, 2018
- F.M. Brunbauer et al. The planispherical chamber: A parallax-free gaseous X-ray detector for imaging applications, NIM A, 2017